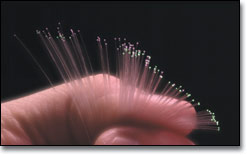
The most challenging task in photonics assembly is the alignment and positioning of optical fibers and components. Submicron accuracy, fine resolution and high stability are required when coupling laser light with the core of an optical fiber. This delicate process is referred to as active alignment. Traditional active alignment involves coupling a light source, such as a laser, with one end of an optical fiber to determine the optimal position of the component at the other end of the fiber.
Manufacturers of lasers, amplifiers, connectors, filters, receivers, switches and other fiber optic components and modules are very concerned about the amount of signal loss that occurs across the component-to-component or component-to-fiber junction. Due to the extremely small size of optical fiber--8 or 9 microns for single-mode fiber cores, which is less than the width of a human hair--very high-resolution systems capable of producing nanometer-scale displacements are required to reduce losses across the optical junction.
If alignment of the optical fiber and component is off just slightly, the product will be useless. With packaging accounting for one-third to 50 percent of the cost of optical components, that's something manufacturers simply can't afford.
Fortunately, a wide variety of manual, semiautomatic and automatic nanopositioning devices are available. Nanopositioning refers to precise motion and positioning with the accuracy of a fraction of a micrometer.
Fiber is measured in microns and wavelengths are measured in nanometers. A micrometer--also called a micron--is one millionth of a meter. A micron is equal to 10-6 meters. A nanometer is one billionth of a meter. A nanometer is 1,000 times smaller, or 10-9. One micron equals 0.00003937 inch, while 1 nanometer equals 0.00000003937 inch.
With assembly requirements ranging from 10 microns down to submicron levels, precise alignment is critical to achieving accurate and consistent results. "Amplification of light signals is very important," says Terry Caves, vice president of engineering and manufacturing at Zenastra Photonics Inc. (Ottawa, ON). "Space is always a premium, because the work envelope is in the tenths of microns."
The primary function of nanopositioning in photonics assembly is to align transmitting and receiving components to minimize light loss in optical couplings. Currently, optical coupling tolerances are 0.1 micron. "As photonics technology advances, these tolerances will become increasingly tighter," predicts Sarah Cremer, associate director of marketing at NEXT Fiber Systems (Cambridge, MA).
"What assemblers are lining up is the core of the fiber," says Jim McEleney, vice president of sales and support at kSARIA Corp. (Wilmington, MA). "Any misalignment will result in a loss of signal. Misalignment of only one-tenth of a micron will result in a 30 percent loss of light coupling. Optical fiber is not like copper wire, where you can be off in your alignment or connection and still transmit electricity."

Numerous Challenges
The delicate and unpredictable nature of optical fiber creates numerous challenges for assemblers. "During the alignment process, there are many different variables to take into account," says McEleney. "For instance, the core of the fiber is not in the same place each time, because of the fiber manufacturing process."Optical fiber is made with a process called the "gob drop," which is very similar to a dripping piece of taffy. A large preform rod of highly purified glass is mounted vertically in a furnace and heated to more than 1,200 C. The bottom tip, known as the gob, melts, separates from the cylinder and falls down a 4-story drawing tower, pulling behind it a thin strand of glass fiber. As it is exposed to air, the hot glass thread emerging from the furnace solidifies almost instantly. A protective coating and cladding is applied and the fiber is wound onto a large spool.
Due to its small size, extreme accuracy and small displacements are required to correctly align fiber. Factors such as curvature of the fiber tip and the gripping position on a fiber pigtail add an extra degree of variability to the alignment process. "Another challenging factor is the lack of standardization in some of the products and techniques, such as packaging designs and attachment processes," says Cremer.
Because optical fiber is so finicky, many photonics assembly processes require up to six degrees of freedom in as small a footprint as possible. "To align things mechanically, you have to look at six different axes," says Caves. "Three linear and three angular motions are required: X, Y, Z, 0x, 0y and 0z."
Another challenge is the unique physical parameter of optical components. For instance, a single-mode fiber has a core approximately 9 micrometers in diameter. "To accurately align this core, an assembler needs a typical positional accuracy of 50 nanometers," says Emilio Saturnino, director of marketing and operations at Luminos Industries Ltd. (Ottawa, ON). "Industry actually demands an accuracy of 20 nanometers." The scale of the devices themselves can vary from the 9-micron diameter of a single-mode fiber to 10 millimeters wide for a waveguide.
Work envelopes vary depending on the type of device being assembled and the overall degree of automation required. "Coarse" motion positioning is used to guide the optical fiber and component into the correct vicinity for final placement and assembly. There are coarse movements on the scale of tens of millimeters that may be required to enable the loading and unloading of parts. However, the "fine" stage of the component alignment process may require motion on the scale of tens of microns.
"Unlike many industrial automation systems, typical photonic assembly workcells are benchtop systems," says Cremer. "Actuators and positioners have strokes less than 1 millimeter."
"The typical envelope is a square 2 to 3 feet in size," adds Saturnino. "The typical limitation for large positioning jigs would be the four corners available on the square projected by the work envelope. This means an assembly station equipped with conventional positioning stages would get very difficult to work with if more than four positioners were required for that particular assembly operation."
"Motion envelopes in some applications, such as assembly for fiber-to-device, are limited to hundreds of microns," says Amir Chitayat, president of Sagitta Inc. (Fremont, CA). "Other applications, such as fiber Bragg grating, are in hundreds of millimeters to meters. In polishing and inspection applications, travels needed are in the 300-millimeter range."
"Many of the motion requirements in the fiber optics field require motion parameters that are contradictory to many standard motion systems," adds Chitayat. "On one hand, there is the need for very high resolution and small steps of 0.05 micrometer, while at the same time the system must achieve reasonable speeds to address the increasing need for higher and higher throughput for assembly and polishing operations.
"This is why many equipment manufacturers are forced to incorporate into their systems many axes in a coarse or fine mode, which only attempt to address the difficult issues at hand. If the manufacturer is not careful to consider all aspects of the system during the up-front design process, the result can be a more complex system, which is both expensive and less reliable."
Manual Methods
Until recently, most fiber optic components were assembled manually in lab-like settings. In fact, hand-operated micrometers still are the workhorse of photonics manufacturing. These positioning devices usually have manual adjustment screws, fine internal threads and an outside scale so that precise displacements can be made and repeated.But, faced with growing product demand, rising labor costs and inefficient yield rates, many fiber optic component manufacturers are desperately searching for automated solutions to traditional manual processes. "The future trend of automation is to completely replace the human element in photonics assembly," says Saturnino. "All manufacturers are moving toward fully automated systems." They are scrambling to automate because they're under intense pressure to boost yields.
However, expensive legacy manufacturing jigs and assembly stations are still common in most manufacturing facilities. "The fiber optics industry has evolved over time and there has been extensive investment in photonics assembly stations that are now not suited for accurate single-mode fiber optic components," says Saturnino.
"Manual, lab-grade equipment can provide several axes of motion in an area that is only a few inches cubed," adds Mike Formica, general manager of the Fiber Automation Div. of Axsys Technologies Inc. (Pittsburgh). "In many cases, these positioners offer inadequate travel or resolution for a production machine.
"There are companies out there that have been selling to the photonics industry for quite some time that offer outstanding footprint, but are not suited for 24/7 production," Formica points out. "Conversely, there is a group of companies that offer highly reliable stages that are entirely too big and bulky. Bridging that gap has proven to be very difficult for many of these companies.
"Most of the lab-grade equipment manufacturers are fundamentally not stage companies and have not created production-grade staging solutions," claims Formica. "Meanwhile, most of the stage manufacturers aren't experts in photonics and don't offer products that address all of the industry needs." According to Formica, the ideal solution is a product that offers all of the features of a lab-grade positioner with a hardened design that is suitable for full-scale production.
There are two basic forms of automation compatible with the unique demands of photonics assembly: operator-assisted motorized systems and fully automated operator-loaded systems. "Achieving the later requires a method of identifying fiber position, either through complex means such as machine vision or by collision detection, which is more rudimentary and less complicated," Saturnino points out.
Of course, automated solutions are not cheap. According to Saturnino, fully or semi-automatic systems typically cost $30,000 to $100,000. "The 'holy grail' would be a system that is loaded with the materials and at the press of a button just goes, and is cheap, compact and very stable," quips Saturnino.
Whether manual or automatic processes are used, submicron positioning and alignment requires repeatability and reliability. "Designing and manufacturing devices capable of repeatable submicron positioning is no small task," says Ron Rekowski, director of product marketing for the positioning systems division of Aerotech Inc. (Pittsburgh). "Absolute accuracy, though important, is not as critical as there is usually a power coupling feedback loop in the system.
"Reliability is important in any automation product designed to operate in a 24/7 environment while maintaining laboratory-grade resolution, accuracy and repeatability," adds Rekowski. "The performance of the equipment must remain stable throughout its operating lifetime to maximize the throughput of the process."
Motion Options
Motors, stages, carriages, slides, tables and controls play a key role in nanopositioning systems. Most motion control devices are based on linear motor technology, piezo technology and rotary motor or ballscrew technology.Hardware is necessary to hold and move workpieces in three-dimensional space, and to limit vibration, backlash and other sources of error in the process. All motion control equipment suitable for fiber optics assembly applications must have a high resonant frequency and high stiffness, in addition to maximum accuracy and repeatability.
Several different types of actuating systems are available. They can be classified by the technology used to create movement and final accuracy of component placement within the system. Typical systems include:
- Flexure stages, where the movement is the result of bending a very rigid structure within the elastic limits of the same structure.
- High precision roller-bearing stages, with glass scale count encoders used in a closed-loop system to create the necessary stability for maintaining the position.
- Piezo-assisted fine-displacement combined with control circuitry and conventional roller-bearing or flexure technology.
- Any combination of technologies using ultraprecise methods for moving very small increments, such as linear motors.
"A piezo system is useless without an encoder to eliminate hysteresis," explains Formica. "Likewise, a linear motor system is totally impractical if the footprint is too large. And for many applications, the best linear system is virtually useless without comparable rotary axes."
"The most critical item in a nanopositioning system is the motor," claims Rekowski. "If you cannot drive the system with the required accuracy and repeatability, you can never create a true nanopositioner."
"Effective nanopositioning requires an optimal combination of the drive, feedback, mechanical system and the servo controller," adds Sagitta's Chitayat. "The recommended drive is either a linear motor or a piezo actuator, which offers very high motion resolution without backlash."
According to Chitayat, the mechanical structure should be stiff and exhibit high natural frequency. "The bearing systems are typically either crossed roller bearings (for short strokes) or low-friction recirculating bearings for longer strokes," he explains. "In some cases, air bearings can be used, which offer very high flatness and smoothness, but are more expensive and sensitive to ambient environments."
Most observers believe noncontact, direct-drive motors provide the best combination of size, speed and reliability for nanopositioning, whether they are applied to linear or rotary motion. However, they disagree about the merits of linear motors and less expensive options, such as piezo-driven flexure stages.
"There's a very distinct trade-off on using each type, based upon the application," says kSARIA's McEleney. "Piezo is the most widely used type of actuator for nanopositioning. Linear motors will probably win out eventually, but they are still the more expensive option."
"Direct-drive linear motor technology provides virtually unlimited travel capability, no wearing elements for consistent performance, and the highest possible speed," claims Rekowski. "All of these features combined provide the highest throughput and the lowest total cost of ownership.
"The linear motor is typically larger, but offers the advantage of higher speeds, forces and acceleration," adds Chitayat. "Such a system should offer direct feedback from the moving element in order to minimize abbe errors. This is typically achieved by using high resolution, non-contact linear encoders or laser interferometers."
"Piezo-driven flexure stages are capable of producing nanometer scale motion, but are limited in travel and exhibit hysteresis and parasitic off-axis motion," says Rekowski. "Piezo friction drives overcome the small travel of the piezo flexure stages, but they tend to be limited in terms of speed, and their performance degrades over time due to the wear induced by the friction-drive element."
Other observers, such as Luminos' Saturnino, believe flexure stages are a better option. "A flexure stage is by far the superior method of translating precision motion," argues Saturnino. "A flexure stage is based on frictionless motion translation, which provides the best repeatability.
"It challenges other technologies out there by being able to place a single-mode fiber in position (alignment) within the tolerable limits of accuracy demanded by single-mode fiber technology and does this without complicated feedback control systems," explains Saturnino. "What this means is that a simple, low-cost motorized positioner will give similar performance stability to a system costing five to 10 times as much."
According to Saturnino, the all-flexure system offers virtually perfect Cartesian motion, with high stiffness and immunity to external influences. "The positioner is specifically designed for precision and stability," he explains. "This makes it very easy to use a low cost actuator, or simply use it as a manual positioner. It tackles the main challenges of photonics assembly--accuracy and stability--head on."
No matter what type of motion control technology is used, the accuracy of the positioning system and its resistance to external influence over time is critical. This is particularly important when curing adhesives used in the assembly process.
"When making submicron incremental moves, it is critical that you do not overstep your target position," warns NEXT Fiber Systems' Cremer. "It is also important that all of the components of a nanopositioning system are integrated and work well together. This includes nanopositioning platforms, software and feedback systems."
Software controls the movement of nanopositioning stages and can be used to improve the accuracy and repeatability of the hardware. "Software also is involved in power searching algorithms used to maximize the coupling efficiency of the optical connection," says Rekowski. "Kinematic transformations may also be implemented in software to enable the programming of the precise motion of the fiber or device through a combination of both linear and rotary stages."
Future Vision
There is an ongoing trend of using machine vision technology for nanopositioning. "As package sizes get smaller, it will become more important to the assembly process," predicts McEleney. "Vision provides positional feedback to the control system."Vision is often used to initially acquire the location of the fiber relative to a device. "In many cases, the production is still largely manual and there is a great level of uncertainty in the position of the various elements," notes Axsys' Formica. "In its most simple form, machine vision can be used by an operator to monitor the process at a high level of magnification. In a truly automated machine, it is used to position the components prior to active alignment, with fiber optic power levels being used for fine alignment."
Machine vision is important, because it minimizes operator input and reduces the complexity of fixturing devices. "With manual inspection methods, it may take an operator up to a minute to thoroughly examine a close-up image of a fiber end for defects," says John Keating, industry marketing manager for fiber optics and photonics at Cognex Corp. (Natick, MA). "And, because the process is highly subjective, operators may visually interpret defects different ways."
"Vision can be used as a tool to bring components into initial alignment much quicker and more reliably than a skilled human operator could using a microscope and manual positioning stages," adds Rekowski. "Using vision to find the orientation and position of components also reduces the complexity and cost of fixturing these devices." Incorporating more and more vision technology into the fiber-to-device attachment process will lead to higher throughput, a more repeatable process and better quality control.