
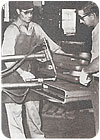
In the late 1950s, no one ever heard of OSHA or the EPA, so this is how welding was done.
When ASSEMBLY first started to cover fusion production methods in 1958, manufacturers typically joined metal parts with bulky arc welding equipment. The manual shielded metal arc welding process was widely used, because gas metal arc welding and flux cored arc welding were still in their infancy.
Resistance welding was the rule rather than the exception. Assembling plastic parts with solid-state welding processes, such as ultrasonics, was unthinkable. Friction stir welding, electron beam welding, plasma arc welding, laser welding, magnetic pulse welding, infrared welding, robotic welding and other processes commonly used today had not been developed yet.
Spurred by the burgeoning aerospace industry, assemblers in the late 1950s were busy experimenting with new ways to weld thinner and thinner sheets of material. For instance, a news item in the inaugural issue of ASSEMBLY revealed how Solar Aircraft Co. (San Diego) was welding metal foil “half as thick as a human hair” into rigid structural panels for aircraft and missiles.
“From one to four needle-like welding heads move up and down the corrugated strips . . . and as many as 240 individual resistance welds are made each second,” the article pointed out. “The machine will continue to add layer upon layer of honeycomb cells until the core has reached its desired width.”
An article in the July 1963 issue of ASSEMBLY pondered what welding system would be the most appropriate for use in outer space. In addition to examining the pros and cons of electron beam welding and pressure welding, the article said, “it is altogether possible that welding could be accomplished through the direct focusing of sunlight.”
The author did not detail how that would be accomplished, but he did point out that “there is no simple answer, since all systems have both favorable and unfavorable aspects. The ultimate answer lies in a careful balance between the system’s capabilities, its limitations and its power requirements.”
Back on earth, manufacturers used welding technology to hold a wide variety of products together. For instance, an article in the November 1958 issue of ASSEMBLY explained how the Victor Adding Machine Co. (Chicago) used resistance welding to join 0.115 inch thick cold-rolled steel components. Welding solved the company’s “requirements regarding dimensions and a stronger assembly.”
In the days before electronic calculators and computers, Victor was a leading manufacturer of mechanical adding machines such as the Comptometer. While technology and history passed the company by, many of its products still work flawlessly, thanks to welding.
Fifty years ago, none of the advanced materials that are available today existed, says Harvey Castner, technology leader for arc welding at the Edison Welding Institute (EWI, Columbus, OH), which was established in 1984 as an independent R&D center. “The space program, nuclear weapons, nuclear power and advanced aircraft fostered the development of advanced materials and processes to join them,” he explains. “This includes high-strength titanium and aluminum alloys, as well as composite and plastic materials.”
Ultrasonic Welding
In November 1960, ASSEMBLY published an article on a relatively new joining technique called ultrasonic welding. Although the technology was invented in the late 1940s, a landmark U.S. patent for the ultrasonic metal welding process was granted in 1960 to Aeroprojects Inc. (West Chester, PA), which later became Sonobond Ultrasonics. The first commercial use was for fine wire bonding in the growing electronics industry.“Ultrasonic welding is one with many possibilities not heretofore practical with other types of welding,” explained the ASSEMBLY article, which was written by an engineer in the semiconductor products division of General Electric Co. (Fairfield, CT). However, he pointed out that “one of the disadvantages of ultrasonic welding is the number of variables involved,” such as tip geometry, frequency, power, time and clamping force. The author included a full-page table to help readers visualize and grasp the overall procedure.
The process of ultrasonic plastic welding started in 1963, when Robert Soloff, founder of Sonics & Materials Inc. (Newtown, CT), was experimenting with an ultrasonic probe. He brought the probe into contact with a plastic tape dispenser and welded its two halves together.
Soloff realized that sound waves could travel around corners and down the sides of rigid plastics to reach the joint area to be welded. He initially tested the process on several toys that had been glued together, such as harmonicas and dolls. Soloff cut apart the toys, contacted the parts with the probe, and welded them together successfully.
When Soloff presented the ultrasonic plastic assembly process to engineers at Ideal Toy Co. (New York), they asked him to replace the handheld method with a more automated approach. He took a drill press stand that he used in his home workshop and modified it to hold a converter and half-inch step horn.
Soloff added an air cylinder, a valve and a couple of mechanical timers, and finally, wiring to turn the ultrasonics on and off with a weld timer, a hold timer and a foot switch. Ideal bought this first ultrasonic press on the spot for a Roy Rogers stagecoach cowboy kit, and a whole new assembly process took off. In 1965, Soloff was awarded a U.S. patent for the ultrasonic method for welding rigid thermoplastic parts.
“No single joining method or design has significantly impacted the popularity and use of plastics,” says Marc St. John, EWI’s senior engineer for plastic welding. “However, in terms of impacting manufacturing, the high speed and versatility of ultrasonic welding-particularly for smaller components-makes it the most common method used today.”
“As plastic replaced steel in many applications, ultrasonic welding [was] called upon to join these parts,” adds Ken Holt, applications manager at Herrmann Ultrasonics Inc. (Bartlett, IL). In addition to automotive parts and consumer goods, the technology is now used to assemble a wide variety of medical devices. Manufacturers of solar panels are also using ultrasonic equipment to weld the aluminum interconnects used between photovoltaic cells.
“What started as a means of assembly for commodity items has evolved into an accepted method for the assembly of highly detailed, close tolerance, costly and critical items throughout the marketplace,” explains Holt. “[Today’s automobiles have] ultrasonic welding applications in most all subsystems, such as electrical and lighting, fuel delivery, braking, air handling, electronic systems, interior sound deadening and appearance items.
In its April 1969 issue, ASSEMBLY reported on the first car that featured a body and chassis made entirely of plastic. The two-door Formacar, developed by engineers at BorgWarner Inc. (Auburn Hills, MI), was assembled with an ultrasonic pistol-grip hand tool.
While that vehicle concept failed to revolutionize the auto industry, ultrasonic welding did. Most applications have been related to joining electrical conductors, contacts and terminals. “The automotive industry has used ultrasonic welding since the 1980s to weld copper to aluminum for ignition modules, wire to terminals, and wire-to-wire for harnesses,” says Janet Devine, president of Sonobond.
“A greater knowledge of the fundamentals involved in ultrasonic welding has led to its wider use, better joint designs, and new product designs that take advantage of the process,” adds Devine. “The invention of more sophisticated and relatively inexpensive electronic devices and controls has made a huge contribution to making the equipment cheaper, when adjusted for inflation, than it was in the 1960s.”
Microprocessors and precision pneumatic components have also made ultrasonic welding easier and more productive in recent years, says Rodger Martin, vice president of sales at Branson Ultrasonics Corp. (Danbury, CT).
Today, automakers are scrambling to reduce the weight of vehicles and increase fuel efficiency. A C-frame ultrasonic welder, which can be operated with a robot arm, is currently being used in the industry to weld large aluminum sheet metal parts.
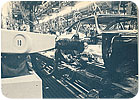
By the late 1960s, automakers started to use robots equipped with spot welding guns.
Robotic Welding
In addition to ultrasonic welding, the auto industry has driven the development of robotic welding and laser welding technology. When ASSEMBLY debuted in the late 1950s, automotive body shops were dominated by large jigs. Welders equipped with face masks, goggles and portable equipment worked inside and outside of vehicle shells to attach steel structural supports, body panels and hundreds of other stamped parts.Although automatic welding equipment was used, the intricacies of most automotive applications required more than 80 percent of the welds to be accomplished manually with portable spot welding guns. Working in cramped quarters, with sparks and fumes flying through the air, it was a dirty, noisy and dangerous task. Ironically, automakers began experimenting with robotic welding at the same time that the Occupational Safety & Health Administration (OSHA, Washington, DC) was established by Congress.
When General Motors Corp. (GM, Detroit) opened its state-of-the-art Lordstown, OH, plant in 1970, more than 90 percent of body welding operations were automated vs. 20 percent to 40 percent at traditional auto plants. It featured 75 automatic welding devices, including 26 five-axis Unimate robots that each performed about 20 spot welds.
“Each can move a welding tool through a predetermined series of motions to provide weld accuracy within 1/16 inch,” reported a feature article in the October 1970 issue of ASSEMBLY. “Each Unimate memorizes the exact ‘point-in-space’ location for each weld position. On command, it accurately repeats the pattern of tool movements and produces 2,000 welds per hour.”
“Early programmable behemoths were large and mostly hydraulic-powered, with limited computing power,” says Mike Sharpe, director of materials joining at FANUC Robotics America Inc. (Rochester Hills, MI). “Today’s handheld PDAs and portable electronic games have more computing power.
“Because of their size, they were primarily used for handling heavier loads and not suited for continuous path applications like arc welding,” adds Sharpe. “Cost also limited their proliferation. Consequently, [automakers] were the initial users and these early adopters used robots for handling a spot welding gun for body welding.”
According to Sharpe, the advent of compact electric servomotors helped manufacturers develop smaller and more man-sized robots with improved compactness and better path control over their hydraulic predecessors. “As a result, the better path performance provided the necessary control for arc welding applications,” he explains. “Electric servomotors were first introduced as a DC brush-type motor and were large in size compared to later developments in AC servomotors.”
Robotic technology has helped make welding cost-effective in terms of speed, accuracy, repeatability and flexibility. When paired with robots, processes such as resistance spot welding and MIG welding were able to join metal parts better, faster and with more consistency. Robotic spot welding is now used to assemble garage doors, metal enclosures, household appliances and aircraft components.
“Robots have influenced welding technology in a very positive manner,” says Rohit Khanolkar, senior engineer at Applied Manufacturing Technologies Inc. (Orion, MI). “The speed, accuracy and repeatability of robots make them indispensable in welding operations in manufacturing.
“Robots have allowed for welding flexibility in terms of welding multiple products through the same line,” adds Khanolkar. “Robots have also made welding applications safer since the welding cells can be isolated with little or no human intervention, making manual welding obsolete, especially in high-speed manufacturing lines.”
In addition to addressing safety concerns and ergonomic issues, welding robots allowed automakers to build flexible body shops. For instance, Toyota Motor Corp. (Nagoya, Japan), which started using hydraulic welding robots in 1971, developed a flexible body system in the mid-1980s that allowed it to produce five different vehicles on the same assembly line.
By focusing on continuous improvement, Toyota engineers refined the robotic welding process. In 2002, the automaker unveiled a global body line that gives it the ability to assemble up to eight different vehicles at a time using the same equipment. The new system operates at roughly half the cost of the old setup, which required expensive downtime for retooling. It positions jigs on the inside of each car body, rather than outside.
Toyota engineers are currently working on a next-generation body welding line at the Takaoka plant in Japan. When the system is complete in 2009, the extremely flexible facility will build more models, faster, on shorter assembly lines than any other auto plant in the world.
Engineers at ABB Robotics (Vasteras, Sweden) recently developed a controller that coordinates the movement of several spot welding robots, allowing them to simultaneously work on the same part or component. For instance, several robots can handle a car body at the same time, reaching below or into the same vehicle at the same time without disturbing each other. The improved flexibility results in greater time savings, while also improving cycle times and throughput.
In the future, welding robots may also be connected with wireless controls that eliminate expensive wire and cable, while improving flexibility. Wireless technology is currently used to control robots at a Volvo assembly plant in Sweden. Communication is accomplished via a wireless I/O module that is attached to SCARA robots.
Laser Welding
After the laser was invented in the late 1950s, manufacturing engineers embarked on research efforts to harness the technology for welding applications. They were attracted to the unique noncontact nature of laser welding, which promised to simplify fixturing and put no mechanical strain on the workpiece. Another benefit was the ability to join both similar and dissimilar materials with a minimal heat-affected zone.Early manufacturers of laser welding equipment included GTE Sylvania Inc., Raytheon Corp. and United Technologies Corp. The first automotive laser welding application occurred in 1976 at GM’s Dayton, OH, plant. It used two 1.25-kilowatt CO2 lasers to join valve assemblies for emission control systems.
Laser welding was the cover story of ASSEMBLY’s October 1976 issue. “Just a few years ago, the laser was held in awe by some and actual fear by others,” the article pointed out. “But, today, it has broken out of the Buck Rogers era, and has come of age as a viable production welding tool.
“Laser welding is becoming less expensive than electron beam welding, which requires that the work be performed in a vacuum chamber-a time consuming process. The laser, on the other hand, operates under normal atmospheric conditions.”
However, despite that initial promise, laser welding technology was slow to catch on due to high costs, safety concerns and reliability issues. Changing market conditions, new materials, new production processes, more affordable laser modules and the emergence of high beam quality lasers, such as disc and fiber lasers, have recently prompted manufacturers to investigate the pros and cons of welding parts with light.
Today, automotive engineers are experimenting with laser welding as they search for a cost-effective way to mass-produce fuel cells. Bipolar plates, fuel processors, reformers, heat exchangers and other fuel cell components require hundreds of thin, stainless steel, sheet metal parts, ranging in thickness from 0.1 to 0.5 millimeter.
Because of heat extremes and safety concerns, welding is the only practical way to join and seal fuel cell components. However, it is also the most time-consuming fuel cell production process.
Stan Ream, EWI’s laser technology leader, says lasers hold tremendous promise for fuel cell manufacturing applications because of their high throughput rates. “It is possible to produce laser welds in thin stainless steels at speeds in excess of 30 to 40 meters per minute,” he points out.
But, Ream claims that today’s laser welding methods are either too slow or too expensive for metallic bipolar plate manufacturing. “Fiber lasers for microwelding bipolar plates need to be developed to achieve linear welding speeds greater than 50 meters per minute,” he explains.
Other Welding Technology
In addition to ultrasonics, robotics and lasers, other technology has transformed welding over the last 50 years. For instance, hot-plate welding, which was developed in Europe during the 1950s, became popular in the United States during the mid-1970s.
An article in the December 1976 issue of ASSEMBLY explained how GM was using the technology to assemble a plastic sphere used as a vacuum reservoir in air conditioning control systems. A valve was attached to one half of the polypropylene injection-molded part by spin-welding.
Hot-plate welding was also being used to assemble battery cases, air conditioning ducting and taillight lenses, the article pointed out. The appliance industry used the technology to assemble parts such as detergent dispensers for washing machines and doors for refrigerators.
Another solid-state process, friction stir welding, was developed at The Welding Institute Ltd. (TWI, Cambridge, England) in 1991. Wayne Thomas, a senior TWI engineer who invented the process, says the first company to apply friction stir welding was Sapa AB (Stockholm, Sweden). It used the technology to produce aluminum extrusions for refrigerators.
In recent years, friction stir welding has attracted the attention of aerospace manufacturers. For instance, it has been used to assemble Delta rockets and fuel tanks for the space shuttle. Eclipse Aviation Corp. (Albuquerque, NM) is currently using the technology to assemble major components of its new very light jet aircraft, such as the cabin, aft fuselage and wings. According to some aerospace manufacturing experts, friction stir welding can reduce joining costs by 20 percent and shorten lead time by 25 percent.
In the future, new forms of welding will continue to be developed. Researchers are currently experimenting with a wide variety of hybrid joining processes to assemble dissimilar materials, such as aluminum, composites and titanium. By combining the best of both worlds, hybrid welding offers new challenges and opportunities.
For instance, at the recent K Show in Dusseldorf, Germany, one exhibitor unveiled a machine that allows end users to perform either pure vibration welding, pure infrared welding, or vibration welding plus infrared preheating. Another hybrid method is weld bonding, which combines the best properties of adhesives and welding. And, hybrid laser welding harnesses the advantages of both conventional arc welding and laser welding technology. A
An article in the December 1976 issue of ASSEMBLY explained how GM was using the technology to assemble a plastic sphere used as a vacuum reservoir in air conditioning control systems. A valve was attached to one half of the polypropylene injection-molded part by spin-welding.
Hot-plate welding was also being used to assemble battery cases, air conditioning ducting and taillight lenses, the article pointed out. The appliance industry used the technology to assemble parts such as detergent dispensers for washing machines and doors for refrigerators.
Another solid-state process, friction stir welding, was developed at The Welding Institute Ltd. (TWI, Cambridge, England) in 1991. Wayne Thomas, a senior TWI engineer who invented the process, says the first company to apply friction stir welding was Sapa AB (Stockholm, Sweden). It used the technology to produce aluminum extrusions for refrigerators.
In recent years, friction stir welding has attracted the attention of aerospace manufacturers. For instance, it has been used to assemble Delta rockets and fuel tanks for the space shuttle. Eclipse Aviation Corp. (Albuquerque, NM) is currently using the technology to assemble major components of its new very light jet aircraft, such as the cabin, aft fuselage and wings. According to some aerospace manufacturing experts, friction stir welding can reduce joining costs by 20 percent and shorten lead time by 25 percent.
In the future, new forms of welding will continue to be developed. Researchers are currently experimenting with a wide variety of hybrid joining processes to assemble dissimilar materials, such as aluminum, composites and titanium. By combining the best of both worlds, hybrid welding offers new challenges and opportunities.
For instance, at the recent K Show in Dusseldorf, Germany, one exhibitor unveiled a machine that allows end users to perform either pure vibration welding, pure infrared welding, or vibration welding plus infrared preheating. Another hybrid method is weld bonding, which combines the best properties of adhesives and welding. And, hybrid laser welding harnesses the advantages of both conventional arc welding and laser welding technology. A
Learn more about welding history by clicking "Web Extra."