Lithium-ion battery electrodes are usually made using a wet slurry with toxic solvents, an expensive manufacturing approach that poses health and environmental risks. To address the issue, engineers at Oak Ridge National Laboratory (ORNL) have developed a dry battery manufacturing process.
It eliminates the solvent, while showing promise for delivering a battery that is durable, less weighed down by inactive elements and able to maintain high energy storage capacity after use. Dry processing also saves factory floor space, as well as time, energy, waste disposal and startup expenses.
Working with Navitas Systems, the ORNL engineers examined how the dry process affects the structure of battery materials and their electrochemical properties. They focused on an electrode dry processing strategy, which involves mixing dry powders with a binder, then compacting the material to improve contact between the particles. This strategy can be applied to both the anode and cathode by focusing on either certain materials or mixing methods.
After Navitas made the electrodes, ORNL measured their electrochemical performance in different conditions over various timeframes. Batteries made using the dry process showed a “superb” ability to maintain their capacity after extended use. They are “highly chemically desirable,” because their structure allows lithium ions to take a more direct path between the anode and cathode. The electrodes are thicker to allow higher energy loading, while reducing inactive ingredients that increase size and weight.
“There are more active materials in the electrode,” says Runming Tao, a chemical engineer at ORNL who worked on the project. “And, even after cycling, it will have few cracks.”
According to Tao, these two advantages reflect a high energy density and good long-term cyclability. The electrode can bend and flex well, demonstrating excellent mechanical strength and the winding capability needed to mass-produce batteries.
The dry process could offer a variety of benefits to manufacturers and the U.S. supply chain. For instance, it’s highly compatible with current state-of-the-art electrode manufacturing equipment, while its reduced environmental impact makes battery plants suitable in more places.
“When you’re looking at the gigascale factories, you’re looking at billions of dollars in order to scale batteries up,” explains Bryan Steinhoff, technical lead and lead researcher on the project for Navitas. “Dry processing can eliminate the coating and solvent equipment currently necessary for large-scale battery production.
“If you can use a dry process instead, you can reduce your footprint by up to 50 percent, saving hundreds of millions of dollars and starting to enable the creation of an infrastructure to replace one that is largely dependent on Asia at the moment,” claims Steinhoff.
The next step in the R&D project is stabilizing the material that attaches the anode components to a thin metal current collector. One goal is to develop or identify a better binder for the dry process, because the current binder is not very stable for the anode environment.
The engineers also want to reduce the amount of carbon black, a material that maintains battery conductivity but detracts from its energy density. They hope to balance the benefits and drawbacks of the thicker electrode. It has the potential for higher energy loading and is easy to roll, but it may provide less power, since the ions have further to travel.
New Algorithm Enables Autonomous Sensing and Movement
Engineers at the University of California, Los Angeles (UCLA) have developed a new computational procedure that allows autonomous vehicles to perceive, map and adjust to their environments more quickly and accurately. It significantly improves their ability to navigate their surroundings in real time.
Lidar technology has traditionally been used to enable vehicles to visualize their operating environment (mapping) and understand how to navigate their dynamic surroundings (location) at the same time. However, there are limitations on mapping accuracy and reaction time as vehicles struggle with massive data processing and computational complexity.
The engineers created an algorithm that addresses those challenges. Direct lidar-inertial odometry and mapping (DLIOM) technology was used to fly a drone around parts of the UCLA campus. The test flights found the new algorithm-embedded vehicle performed 20 percent faster and 12 percent more accurately than those armed with current state-of-the-art algorithms.
DLIOM contains less than 3,000 lines of code vs. a typical algorithm that consists of 5,000 to 10,000 lines. The design allows for minimal programming redundancy and computational complexity, while preserving flexibility for real-world robotics applications.
The algorithm produces precise geometric maps of nearly any environment in real time using a compact sensor and computing suite. It has several key advantages over other current systems, including faster computational speed, better map accuracy and improved operational reliability.
DLIOM features several fundamental innovations that are designed to enhance an unmanned vehicle’s capabilities, such as remembering previously visited locations, utilizing only relevant environmental information, adapting to changing surroundings, correcting blurry incoming imaging from its sensors and processing novel data. DLIOM also expedites the speed with which vehicles operate by combining data collection and processing into a single step.
“When we send next-generation robots to explore other worlds, they will need to handle a range of environments and conditions, as well as establishing their own maps of their local surroundings,” says Brett Lopez, Ph.D., an assistant professor of mechanical and aerospace engineering at UCLA who directs the Verifiable and Control-Theoretic Robotics Laboratory.
“Our new algorithm shows a great path forward to solving latency problems associated with mapping and localization challenges,” claims Lopez. “More immediately, this could help enhance next-generation robots and safer self-driving vehicles.”
In designing the algorithm, Lopez and his colleagues incorporated an inertial measurement unit (IMU) device that measures the gravity and angular rate of an object to which it is attached. By combining lidar scans with IMU, the engineers developed drones that show improved localization accuracy and mapping clarity.
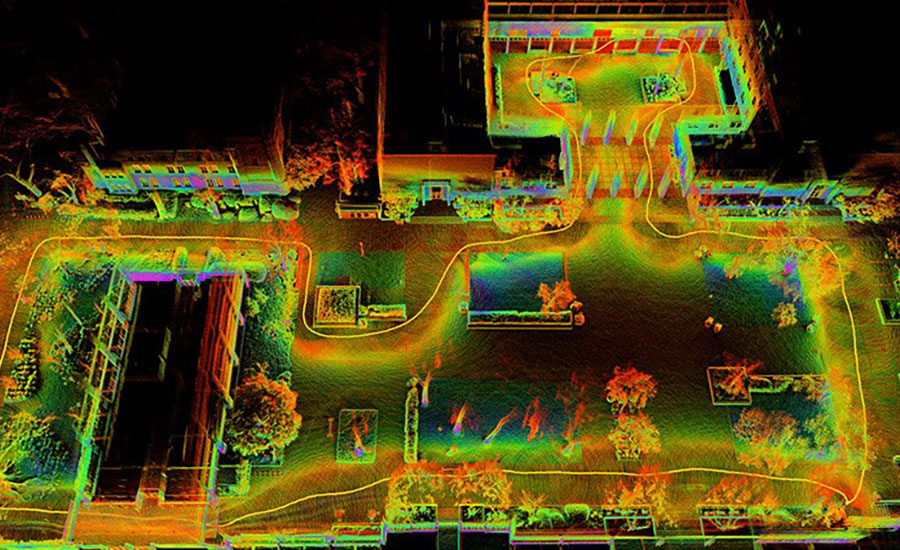
This aerial image was mapped by a drone using new algorithms for improved speed and accuracy. Illustration courtesy University of California, Los Angeles
New Energy Absorption Design Protects EV Batteries
Batteries typically don’t do well in crashes and sudden impacts, which can lead to fires or explosions. To address the issue, engineers from Florida A&M University and Florida State University have developed a new energy absorption design that safely protects EV batteries.
Their design uses tubes filled with paraffin wax, which is a type of phase-change material (PCM). These materials are commonly used to store and dissipate heat, making them useful for protecting batteries from overheating.
“We want to manage the risk of battery damage in a crash,” says Farhad Farzaneh, a doctoral candidate in the Department of Civil and Environmental Engineering at the FAMU-FSU College of Engineering. “This is a significant concern in the overall safety and reliability of electric vehicles and will help advance their adoption in the automotive industry.
“By incorporating PCM-filled tubes in electric vehicle batteries, we hope to prevent catastrophic events and improve the overall reliability and durability of the battery system,” explains Farzaneh.
According to Farzaneh, crash absorbers must be lightweight and capable of absorbing a significant amount of energy during an accident. The PCM-filled tubes soften the blow from impact and absorb heat, keeping nearby battery cells at a safe temperature and protecting against temperature rise that might lead to a fire.
Farzaneh and his colleagues examined thin-walled aluminum tubes with a range of diameters, thicknesses of exterior metal and end cap designs. They developed models to predict their performance according to those parameters and verified those models through experiments.
The engineers discovered that tubes that were capped on their ends and tubes that were filled with PCM absorbed about 43 percent and 74 percent more energy, respectively, than unfilled tubes.
“Impact loading on the battery module is a major risk in adopting electric vehicles,” says Sungmoon Jung, Ph.D., a professor of structural engineering at Florida State. “Of course, every measure you adopt to protect a vehicle has trade-offs for things such as weight. [Our] research found an innovative way to combine two protective measures into one to improve the safety of electric vehicles.”
Besides making batteries safer in the event of a crash, Jung believes that the breakthrough could indirectly improve battery life by minimizing potential damage from a less intense impact or thermal issues.
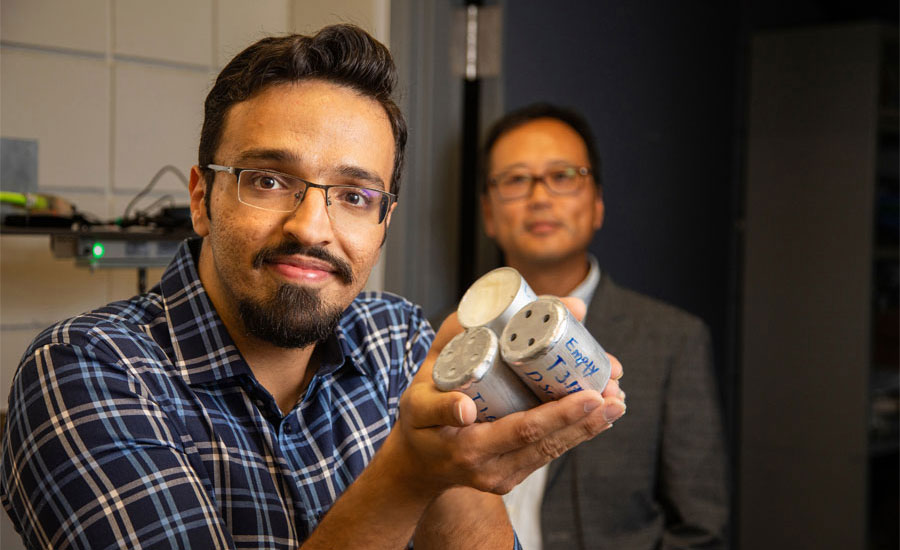
These tubes protect electric vehicle batteries from overheating and impacts. Photo courtesy FAMU-FSU College of Engineering