One of the hardest working types of autonomous vehicles is also the least visible. These extreme AVs work underwater and are designed to stay submerged for days or weeks at a time.
The mini subs play a pivotal role in deep sea exploration. They’re used for a variety of applications, including archaeology, construction, defense, environmental monitoring, farming, inspection, mapping, maintenance, mining, research and surveillance.
These diverse market uses are reflected in the multiple design concepts and range of organizations designing and building autonomous underwater vehicles (AUVs) and unmanned underwater vehicles (UUVs).
The burgeoning market has attracted attention from innovative startups as well as legacy defense contractors such as Boeing, General Dynamics, Lockheed Martin, L3 Harris, Northrop Grumman, Raytheon and Saab.
AUVs and UUVs can be less expensive to procure than crewed ships, because their designs do not require incorporation of spaces and support equipment for people, ultimately reducing life cycle costs.
However, they must be able to collect data, execute tasks and operate efficiently in challenging environments. That’s forcing engineers to tackle numerous design and construction issues to maximize their functionality and efficiency. These challenges range from structural integrity and energy management to sensor integration and navigation precision.
"As the technology has matured and as the market for these vehicles has grown, there's been a lot of players entering it," says Scott Savitz, a senior engineer at RAND Corp., a nonprofit think tank, research institute and consulting firm. "It's also an area where some of the larger traditional defense companies have advantages and [offer] some relevant experience.
"It's a bit like the electric car industry, because while you're seeing the big names, you're also seeing lots of competitors emerge, some without a background that’s explicitly in this area," explains Savitz.
Operating in Harsh Environments
Communications, navigation, endurance, reliability, hydrostatic pressure and material performance are all key design challenges for these types of vehicles.
Materials used to construct AUVs must survive harsh subsea environments and exhibit high corrosion resistance. The entire system, including electronics and navigation devices, must be capable of repeatedly operating at depths with high pressures, including the extra stress that puts on systems.
"As we saw with the recent Titan disaster, if you're sending something to a substantial depth and then it gets exposed to a lower pressure when it ascends again, it will often acquire microscopic cracks or experience subtle damage not easily discerned," warns Savitz.
It's critical to ensure an AUV can handle those changes, along with the capability for the external sensors to withstand the environment.
"Saltwater is very corrosive," explains Savitz. "Some waters have a much higher content of suspended solids than others. Are those going to clog various critical components of the system? How does it deal with grit?"
High-strength materials are typically required to minimize weight and the resultant buoyancy required to keep AUVs neutral—meaning the vessel doesn’t sink or float--in the water column.
Materials must be able to withstand high-pressure environments. That’s why highly noble metals, such as high-nickel alloys, stainless steels and titanium, as well as naval bronze and certain aluminum alloys, are often selected for components.
Carbon-fiber composites are less popular, since AUVs operate at significant depths in the ocean, where water pressure increases dramatically. Carbon fiber structures may not provide the required structural integrity to withstand these extreme pressures without additional reinforcing layers or complex engineering.
However, even with highly noble materials, corrosion control is a significant design factor, so surface treatments and other corrosion mitigation schemes are important.
Engineering thermoplastics also play a significant role because of their strength, low density and immunity from corrosion. Syntactic foam is a unique material that's widely used for its floatation characteristics and high bulk modulus, or low compression at deep depths. Complex shapes can be molded or machined to fit within available spaces inside the AUV form and offset the submerged weight of the vehicle.
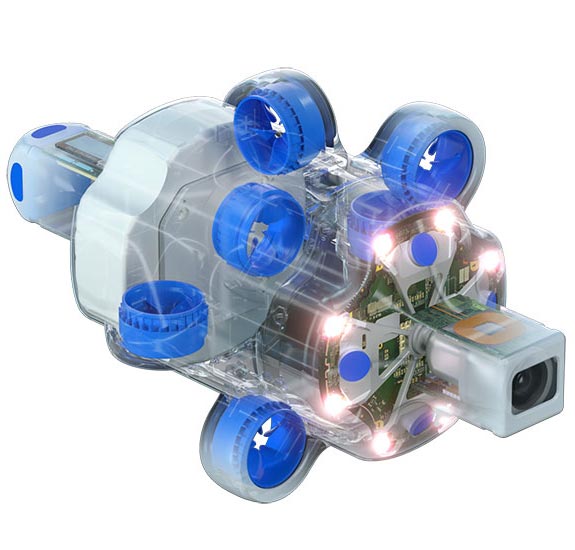
Unmanned underwater vehicles rely on pressure-tolerant electronics. Illustration courtesy Advanced Navigation
In addition, underwater vehicles must be equipped with a variety of sensors that enable them to be operated remotely, semiautonomously or autonomously.
Full, advanced autonomy allows AUVs to operate for months at a time in open, congested and contested waters, with little-to-no human intervention. They can be often accommodate different modular payloads that enable them to be adapted to a variety of tasks.
With flexible, modular payloads, AUVs can accommodate numerous mission sets, and AUV deployment offers a more distributed fleet architecture. That means a mix of vessels and capabilities spread over an increased number of platforms, resulting in a more wide-ranging mission set.
New Assembly Techniques
In Australia, Advanced Navigation recently opened a state-of-the-art subsea robotics manufacturing facility in Perth designed to mass-produce AUVs. This includes the Hydrus, a fully autonomous and compact underwater drone with a depth rating of nearly 10,000 feet and a range of 5.6 miles, equipped with a 4K video camera. The 260-millimeter long device weighs 6.7 kilograms.
The Hydrus is packed with a Doppler velocity log, an acoustic sensor that estimates velocity relative to the sea bottom, an ultra-short baseline acoustic positioning system, an inertial navigation system, and an acoustic and optical modem.
"We saw the rapid development of drones become affordable consumer-grade products, but we didn't see that happening in the subsea robotics space," says Peter Baker, subsea product manager at Advanced Navigation. "We saw that as a market opening for us.
"One of the key success factors for the Hydrus is our manufacturing philosophy,” claims Baker.
The company developed a vertical integration strategy where within one facility engineers develop, manufacture and test almost every single component that goes into the vehicle.
"While it is quite small, it's a very complex piece of technology with multiple subsystems that we built from scratch simply to make the vehicle operate with the level of reliability we require," Baker points out. "We tried a lot of things that were on the market, and we weren't getting what we needed, so we went down the pathway of developing everything in-house."
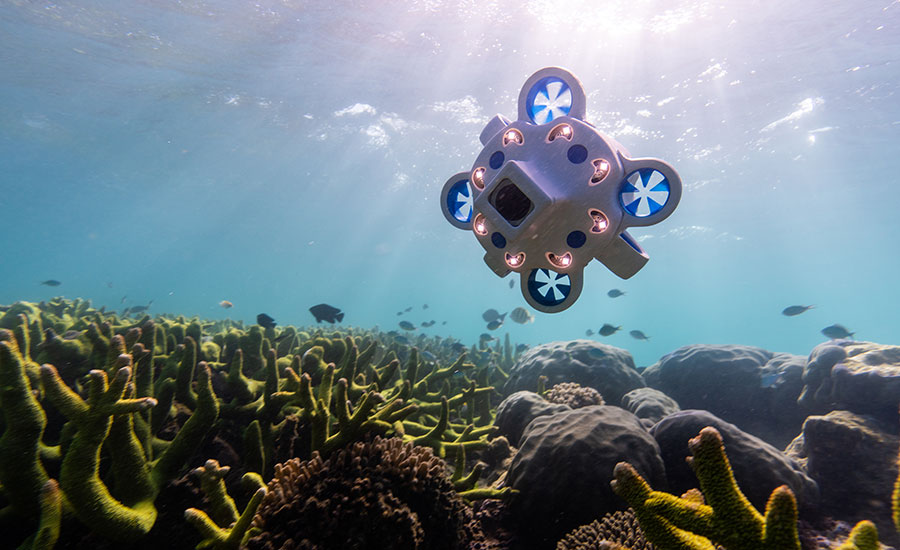
Communications, navigation, endurance, reliability, hydrostatic pressure and material performance are all key design challenges of UAVs. Photo courtesy Advanced Navigation
The ability to share resources between developers and production teams allows the company to accelerate the development process and reduce its reliance on suppliers.
"By taking the innovations we have in the development space and putting that into production quickly, we benefit from that vertically integrated technique," says Baker. "The other side of it is scalability."
Traditionally, most AUV production involves small batch units, with a high degree of hand assembly by skilled technicians.
"We're going more for a much more scalable [assembly] approach--we want to build vehicles in the hundreds of thousands," notes Baker. "To do that, you need a much higher level of automation on the production line."
Baker believes that will drive the unit cost of the vehicles down to make them more accessible to customers that can't afford the other systems that are on the market.
"It also just brings a higher level of quality--the system is not just designed to be a great robot, it's also designed for manufacture," says Baker. "Our R&D developers work with the design for manufacture engineering team."
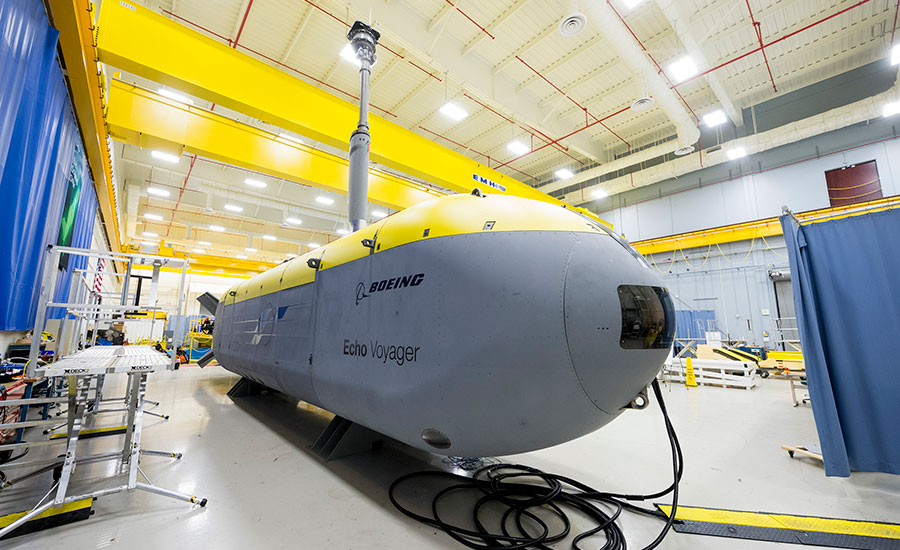
The Echo Voyager is equipped with advanced undersea navigation technology. Photo courtesy Boeing
Pressure-Tolerant Electronics
Advanced Navigation relies on pressure-tolerant electronics. A conventional underwater robot tends to be a large pressure vessel---usually a titanium tube—that is then filled with electronics and sealed up.
"Ttitanium is very expensive, but to keep it neutrally buoyant, the hydrodynamic profile you need to push through the water gets quite big," says Baker. "This means you need bigger thrusters, bigger batteries and the vessel becomes quite large."
Through pressure-tolerant electronic design, all the components are designed in-house with a development technique allowing the circuit boards to be exposed to ocean pressure and ocean water without having any kind of failure.
"That means we can shrink the design down, because we don't have to encapsulate the whole vehicle in titanium," says Baker. "That's the key to the miniaturization of the whole product."
Hydrus bodies are made with injection-molded plastic with interior components made from urethane resins to meet pressure tolerances needed to keep out water and salt.
"During production, we put the product through multiple stages, including a technique that extrudes air out of that resin so that it maintains its integrity," explains Baker.
All the different components are cycled down to the design depth the product is designed to operate at to make sure that they're not experiencing any kind of water ingress or any kind of deformation.
Advanced Navigation produces about 10 AUVs a week. It uses automation to assemble printed circuit boards and other components, which reduces the need for hand soldering and the potential of human error.
"We put components through very high pressures, so we know they're going to work when they go underwater,” notes Baker. "There are opportunities to automate a lot of those processes, such as the testing phase.”
“Efficiencies through automation can also be found on the calibration side,” says Baker. “Because of the numerous high-end navigation sensors installed in each product…we get clear, repeatable results, which leads to a higher level of integrity…without having operators actually input the results.”
As AUVs develop more advanced capabilities, it will be possible to deploy swarms of them to work in a coordinated fashion, surveying larger areas and completing more complex tasks, such as surveying a region for potential wind farm installations or mapping reefs.
"One of the exciting things about Hydrus is that it can live underwater," notes Baker. "It can recharge and transfer data from a docking station. The robots can live ‘in the field’ and collect data as often as the user wants them to."
Swarm capability means the UAVs can communicate with themselves and share a mission plan, a concept called goal-based autonomy.
"You can define a goal you want achieved, and the robots are smart enough to communicate with each other and work together," explains Baker.
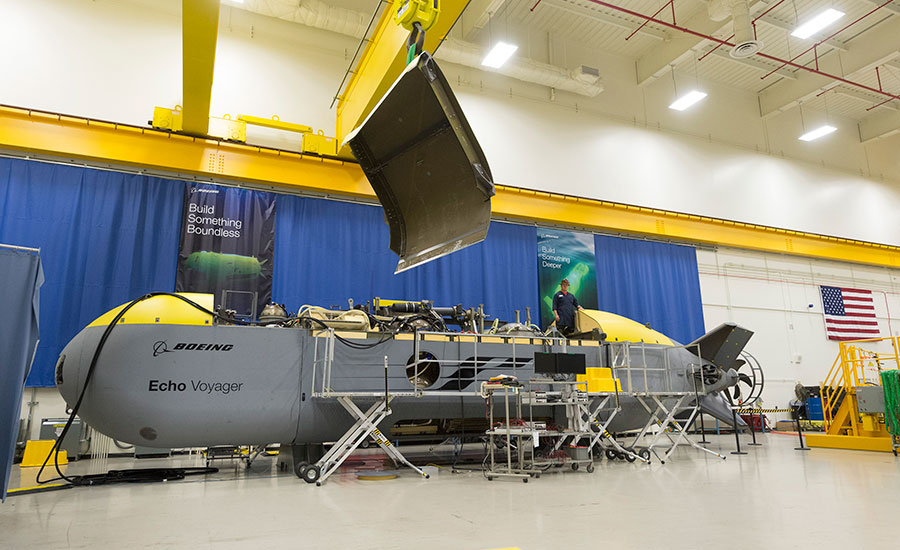
The Echo Voyager is assembled with a variety of fault-tolerant architectures that enable it to work under extreme conditions. Photo courtesy Boeing
Boeing Goes Deep
Boeing has more than 60 years of experience designing, developing and operating maritime systems. In fact, it designed and built the very first uncrewed undersea vehicle, the Special Purpose Underwater Research Vehicle, in 1957.
More recently, Boeing engineers have created extra-large uncrewed underwater vehicles (XLUUVs) such as the Echo Voyager and Orca, which are 51-feet long. The large vehicles were assembled in Huntington Beach, CA. Boeing is also working on a project for the U.S. Navy that involves advanced undersea prototype vehicles designed to meet classified requirements.
The Echo Voyager doesn’t have to be launched from or recovered by a support vessel. Nor does it require a support vessel for operation.
"The vehicle is powered by a hybrid combination of battery technology and marine diesel generators and can also accommodate external payloads," says Ann Stevens, vice president of maritime and intelligence systems at Boeing.
It uses encrypted Inmarsat IV, Iridium, Wi-Fi and FreeWave-enabled communications for operational command, control, system status and mission replanning during near-surface operation. Acoustic communications are used for command, control and status messages during submerged operations.
The vehicle also features a Kalman-filtered inertial navigation unit, Doppler velocity logs and depth sensors. In addition, it can use seafloor long baseline transponders, with GPS available for operating on or near the surface.
"Certain applications do not permit human monitoring or intervention over extended periods, making endurance and reliability key design factors," says Stevens. "Designs must also be readily maintainable."
That means building a UUV to meet these challenges requires fault-tolerant architectures that span software, electrical and mechanical disciplines.
The Orca is a fully autonomous diesel-electric submarine built for a variety of missions with a large payload capacity and energy endurance, which Stevens says pushes the parameters of what is possible in current uncrewed undersea systems.
The Orca design features a modular construction to accommodate an additional 34-foot payload module with an 8-ton capacity, extending its total length to 85 feet.
This versatility allows the Orca to adapt for various missions, including surveillance, submerged and surface operations, electronic combat and minesweeping.
It uses a hybrid lithium-ion battery system for underwater propulsion and diesel generators for recharging when surfaced. The unmanned submarine boasts a maximum speed is 8 knots with a typical service speed of 3 knots, giving the vehicle a range of up to 6,500 miles and an endurance of several months.
"The surface and subsea environments UUVs are exposed to require designs which are robust enough to withstand wide ranges of external pressure, temperature and aggressive corrosion environments, plus extended periods without external communication or navigation inputs," says Stevens.
According to Stevens, the assembly process for a UUV will vary by design, though typically electronics are housed in dedicated capsules, or pressure vessels, within the vehicle’s outer form.
"Electronic assemblies are built and tested, capsules are sealed, and wires are passed through the capsule via special wet-dry interface connectors," says Stevens. "Depending on the architecture, the capsules are then interconnected and tested further."
Vehicle structure design also varies, but typically relies upon a bolted, metallic skeleton overlaid with metallic or thermoplastic skin panels.
With the structure assembled, electronics capsules, syntactic foam, sensors and cabling can be installed. As production scales up, Boeing is looking at leveraging proven, efficient aircraft assembly techniques that utilize robotic drilling.
"These techniques could be readily applied to [our] UUV designs, substantially reducing production cost," notes Stevens.
Other novel approaches that may improve future assembly processes include printed metal and plastic components.

The BIOSwimmer has an aluminum chassis, a foam exterior and aluminum pressure vessels inside to hold the electronics that power autonomous systems. Photo courtesy Boston
Swim Like the Fishes
Boston Engineering Corp. copied nature for its AUV. The BIOSwimmer applies the dynamics of fish—a tuna, to be precise—for operation in constricted areas inaccessible by other vehicles. Developed for the U.S. Department of Homeland Security, the device can inspect a vessel’s exterior for illicit cargo and contraband, as well as harbor facilities for unwanted activity.
"If you look at standard AUVs, you can see that they're pretty rigid throughout the entire body," says Mike Rufo, vice president of research and development at Boston Engineering. "We leveraged biology—there are no torpedo-shaped fish out there—to figure out what we would need for getting into tight areas that another design with a larger turn radius couldn't get into."
The unique design also improves maneuverability and improves hydrodynamics, reducing the power requirements needed to keep pace with a moving vessel.
"One of the other things biological systems provide is what we call a transient response, which is the ability to shift directions and speeds very quickly," explains Rufo. "If you can leverage how efficient biological shapes are in the water, you can operate more efficiently and do it for a longer period of time."
The BIOSwimmer has an aluminum chassis, a foam exterior and aluminum pressure vessels inside to hold the electronics that power the autonomous systems and provide the desired depth rating. It uses a flooded system where water passes freely through the vehicle, plus a modular design.
"We can make the individual modules themselves larger, or we can extend the frame so that you can add additional modules in there if you wanted to add a new sensor or more batteries," says Michael Conry, a mechanical engineer at Boston Engineering who worked on the project.
"There's a big push in underwater space for increased autonomy,” adds Conry. “Our vehicles are set up to integrate with other autonomous solutions to provide increased autonomy moving forward."

This hydrogen fuel cell powers long-range autonomous underwater vehicles. Photo courtesy Cellula Robotics Ltd.
Modular Architectures
Canadian AUV manufacturer Cellula Robotics Ltd. has taken a modular approach to its Solus family of long-range XLUUVs that can be customized to meet specific mission objectives and can carry either military or commercial payloads. Additional energy modules can also be added to enable the vehicles to travel further distances to accomplish mission objectives.
The company’s Solus-LR is a 28-foot long-range hydrogen fuel cell powered AUV that boasts an operational range of more than 1,240 miles. It’s designed for port-to-port missions.
An even larger version called the XR is currently under development. The 39-foot-long device will be capable of cruising more than 3,100 miles.
Because they’re modular, the Solus UAVs can be reconfigured for different missions and payload requirements.
"There could be a particular thing a customer wants to observe on the seafloor, or they might be making a map of the seafloor with sonar vs. lidar," says Andrew Ronan, director of engineering at Cellula Robotics. “They will want to add additional payload modules onto our vehicle and we can extend the length quite easily."
The Solus XR is designed with two modular payload bays—one toward the nose of the vehicle and one toward the tail—that can be swapped in in a matter of minutes.
The outside of the UAV is fiberglass, which is easy to patch and repair. Holes can be drilled almost anywhere in the vehicle to pass through cables or have sensors protrude from the vehicle without impacting strength or integrity.
Inside the fiberglass shell are the modules containing the electronics, which are designed to withstand seawater pressure.
"Most of the hull on Solus is free flooding, so water will just come in through holes in the skin and balance the pressure across it,” explains Ronan. “It’s only those electronics enclosures that are inside the skin that hold back water pressure.
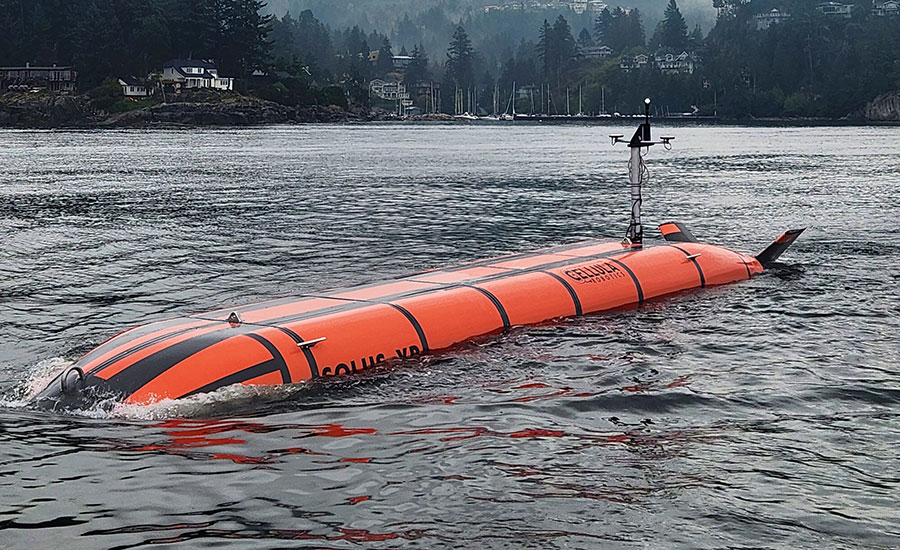
The 39-foot-long Solus XR will be capable of cruising more than 3,100 miles. Photo courtesy Cellula Robotics Ltd.
The XR and LR are both built around a welded aluminium core structure, which gives the vehicle its backbone strength. Core structures are made by third-party suppliers. At Cellula Robotics' facility in Burnaby, BC, subsystems and components are bolted onto the frame, adding significant weight to the vehicle, which should be close to neutrally buoyant. That's accomplished with syntactic foam, a mixture of tiny glass spheres filled with gas and then a resin binder to hold it all together.
"Those blocks tend to be quite large, so often they are put into place early on," says Ronan. "They get bolted into the frame, and while we're assembling the main structure of the vehicle there's quite a few subsystems that [we make]."
"There's a lot going on in the facility simultaneously because of all the different subsystems, including rotary actuators that let the vehicle maneuver, plus thrusters and propellers,” Ronan points out.
Once they've passed their individual tests, they arrive at the vehicle assembly area where technicians install them in the vehicle and connect cables between them.
"[At this point], the vehicle will become more and more complete and start to look more like a submarine," says Ronan. "Once all the parts are in there, we start to move through a very structured testing program, where we slowly start to turn on subsystems and run tests."
Components found on the communication mast of the LR and the hydroplanes on some vehicles are manufactured with metal and plastic 3D printing technology process.
"In its simplest form, just the AUV with a single payload bolted inside, takes about two months to put together, if all the components were there in a pile," says Ronan.
Although AUVs are typically untethered, some lines are used, especially in applications in extremely deep water or when real-time control and data access are critical. Some AUVs are connected to a surface vessel by a cable that provides continuous power and data transmission.
"Some AUVs can be equipped with buoy systems that provide intermittent communication," notes Ronan. "The AUV surfaces and deploys a buoy that establishes a communication link with a surface station or satellite. This allows data transmission and control without a continuous tether."
The choice of whether to use a tether or buoy system depends on the specific mission requirements, depth of operation, communication needs and available technology.
Untethered AUVs are more versatile and can cover larger areas, while tethered systems provide more precise control and real-time data access, but have limited mobility.
"If the AUV is gone for 30 days, part of its mission would likely be to surface every so often and get a satellite link, phone home, transfer the data that it has collected, provide health updates, download a new mission and then continue on," says Ronan.
Learn about new products for autonomous & electric mobility
Articulating Roller Forming Machine with 3-Axis Servo Technology
EBAC – Reliable moisture control solutions for battery manufacturing.
Robot Transfer Unit
Automation for battery manufacturing with Essentials
Robot Integration with Promess EMAP
Zimmer Group – development partner for battery production
New High Performance Controller
ACE Controls offers innovative and flexible solutions