Ultrasonic welding is at work on assembly lines every day, reliably joining a wide variety of plastic and metal parts.
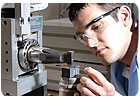
Ultrasonic welding offers assemblers great flexibility and rapid cycle times for joining many plastic or metal parts. Ultrasonic plastic welding is one of the most widely used processes for joining thermoplastics. Ultrasonic metal welding is increasingly being selected for applications where other welding processes are limited, especially for challenging applications where dissimilar metal parts must be joined.
Ultrasonic energy has been used in materials joining processes for a number of years. Ultrasonics were first used for metal grain refinement in the 1930s, followed by soldering and resistance welding enhancement in the 1940s, and then to welding plastic and metal parts in the 1950s.
Ultrasonic plastic welding is very popular for quickly joining semicrystalline and amorphous thermoplastics and thermoplastic composites. It is not applicable to thermoset materials. Because this joining process is versatile, economical and fast, it is popular in nearly every industry for a wide range of applications from children’s toys to medical devices.
Ultrasonic plastic welding is a forge welding process, heating the parts so they soften and flow to produce a weld. In contrast, ultrasonic metal welding produces solid-state welds without melting the base metals. Ultrasonic metal welding has been applied extensively to joining soft materials, such as copper and aluminum, for applications ranging from electronics to aerospace and automotive.

A 40-kilohertz ultrasonic plastic welder joins the cover to the base of an automotive component.
Welding Metal Parts
Ultrasonic metal welding applies mechanical vibrations parallel to the joint interface that create a scrubbing, friction-like relative motion between two surfaces that are held together under strong force. The motion deforms, shears and flattens local surface asperities, dispersing interface oxides and contaminants to create intimate metal contact between the surfaces thereby forming the solid-state weld.Applications of ultrasonic metal welding are extensive, with uses in the electronic, automotive, aerospace, appliance, and medical products industries. Although nearly all metals can be welded with ultrasonics, typical applications involve various alloys of copper, aluminum, magnesium and related softer metal alloys, including gold and silver.
A number of dissimilar metal combinations, such as nickel to copper or aluminum to steel, are readily welded with ultrasonics. Welds can be created between plates, sheets, foils, wires, ribbons and opposing flat surfaces. A common use is joining several stranded copper wires or welding stranded copper wire to a terminal. Ultrasonic metal welding is increasingly used for structural components in the automotive and aerospace industries.
Advantages and Disadvantages.The widespread use of ultrasonic metal welding can most easily be attributed to its advantages. Ultrasonic metal welding is a solid-state, low-heat process. It is excellent for welding high thermal conductivity metals such as aluminum and copper, where the use of resistance spot welding is limited. The process is commonly used in applications involving multiple layers, dissimilar thicknesses, dissimilar materials and welds through contaminants and oxides.
Weld cycles are fast, typically under 0.5 second, and the process is easily automated. Consumables such as filler metal or shielding gas are not required. The energy needed to weld high-conductivity materials is low, especially when compared with fusion welding processes. The ultrasonic welding process can be very repeatable, well within six sigma. However, inconsistent parts will lead to a process that appears to be uncontrollable and inconsistent.
Ultrasonic metal welding does have limitations. Joints are typically limited to lap joints. Although nearly all metals can be ultrasonically welded, the thickness that can be welded is limited by the power supply and metal hardness. Generally, the thickness that can be welded is inversely proportional to hardness. Ultrasonic welding is commonly used for automotive-gauge aluminum alloys and recently has been proven applicable to high-strength steel in similar thicknesses.
Because it is a solid-state process, some amount of deformation is required to disperse contaminates and oxides. While the welding frequency is above the audible frequency range, parts may resonate at a lower frequency that is audible.
Tooling Concerns.Ultrasonic metal welding tooling applies mechanical vibrations parallel to the plane of the joint interface. The tooling includes the welding tip and the anvil, which usually have shapes specific to the parts being welded. The welding tip may be a feature machined directly into the sonotrode or horn, or the tip may be a separate component bolted onto the sonotrode. The parts to be welded are clamped securely between the tip and the anvil so that the ultrasonic energy is concentrated at the interface between the two parts.
The clamping force is quite high and the tooling must be rigid to transmit the ultrasonic energy effectively through repeated weld cycles under the applied clamping force. In addition, although ultrasonic metal welding is a low-heat process and the parts are not melted, the joint can glow red hot during the weld cycle when welding high-strength metal. The tool material must resist bonding to the work during welding.
Sticking between the tooling and the work is traditionally a challenge with ultrasonic metal welding. Consequently, tooling requirements are very stringent. Tooling must have high hardness to avoid deformation, good toughness to avoid fracturing, and good high-temperature strengths.
Variations.The most common ultrasonic metal welding systems produce localized ultrasonic welds, akin to spot welds. There are two distinct systems for applying ultrasonic energy to the joint, called lateral-drive and wedge-reed, but the vibration mechanics at the weld are identical.
The closely related process of ultrasonic microbonding-more specifically ultrasonic wedge bonding-is essentially small-scale ultrasonic spot welding used for electronic interconnects. Torsion welding is also similar to spot welding, but in this case the transducers are arranged so that the mechanical vibrations are angular instead of linear. Thus the tool motion on the part is rotary instead of linear. The torsional weld is therefore circular in nature, and well-suited for applications such as welding copper and aluminum lids on cylindrical containers.
Seam welding uses the same technology as lateral-drive spot welding, but the tool is a solid wheel that rolls along the joint surface. Hermetic sealing of foil bags is a common application of this technology. A new technology, called ultrasonic additive manufacturing, is a variant of the seam welding process. This process welds layers of foil onto themselves, one at a time, to build-up a solid structure. This rapid prototyping process incorporates a CNC mill to machine featuresin situ. Applications include plastic injection molds, metal composite armor and corrosion repair of aerospace structures.
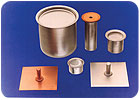
The torsional ultrasonic metal weld is circular and well-suited for applications such as welding copper and aluminum lids on cylindrical containers.
Welding Plastic Parts
Ultrasonic plastic welding applies mechanical vibrations perpendicular to the joint interface, which generates heat via intermolecular friction between surface asperities at the joint interface. When this heat raises the temperature at the interface above its softening temperature, the polymer begins to flow, resulting in a fusion weld after vibrations have ceased.Both amorphous and semicrystalline thermoplastics can be joined using ultrasonic plastic welding, but thermoset polymers cannot. Amorphous polymers have polymer chains that are randomly oriented and intertwined, giving rise to softening over a wide temperature range. Amorphous polymer chains are able to diffuse and flow above their glass transition temperature.
Advantages and Disadvantages.Ultrasonic plastic welding is typically used on parts that are too complex to be molded as one piece. Fast weld cycle times make this process ideal for production. This process is one of the most versatile as the same equipment can weld different parts and thermoplastic materials. Modern ultrasonic equipment provides sophisticated control and monitoring features making the process easy to automate and control, while still maintaining an economical edge over many competing welding processes.
Ultrasonic plastic welding does have disadvantages. There is a high interdependence on process parameters such as power level, weld force, weld time and dwell time. Material properties such as melting point, melt viscosity and modulus all affect the quality of the joint. Not all part geometries are weldable and specific joint designs are required. The dimensional tolerances of molded parts can become critical.
Semicrystalline polymers have regionally oriented molecules. These ordered structures require high heat input to separate and they don’t flow until heating to their melting temperature. More ultrasonic energy is required to melt plastics with higher melting or glass transition temperatures. Semicrystalline polymers tend to dissipate energy instead of absorbing it at the weld interface. Generally, higher amplitude-more power-is required to weld polycrystalline polymers than to weld amorphous polymers.
Fixturing and Joints.Securing the parts in place is critical to success in ultrasonic plastic welding. In fact, the fixture may be the most important element of ultrasonic welding, and its design should not be overlooked. This can be especially challenging in applications that require the use of clamps for quick loading and unloading.
Ultrasonic plastic welding requires specific joint designs to concentrate the ultrasonic energy at a small area to efficiently and quickly promote polymer flow. Prior to welding, the parts should be in contact and able to vibrate. During welding, the joint collapse must be controlled. Close attention to dimensional tolerances is required. The joint design depends on part requirements, type of thermoplastic and part geometry.
Many techniques have been used to improve weld consistency. The most popular method is to use an energy director, which is a triangular protrusion that is molded onto the top weld surface. During welding, the angled point of the energy director has the greatest cyclic strain to produce a high level of heating and promote melting.
As the energy director melts under pressure, it flows outward. As the energy director spreads across the weld interface, it contacts cooler surfaces resulting in a reduction in heating rate. This slows both the melting rate and the displacement. Eventually both part surfaces are in contact and the melting rate increases pushing more melt out of the weld joint. This promotes entanglement of polymer chains across the weld interface and creates a strong bond. The welded part cools under pressure as the vibrations stop.
The energy director is just one of several joint designs that are used in ultrasonic welding. Another popular approach is to rely on an interference fit and shearing effects to concentrate ultrasonic energy on a small area. The melt then helps generate additional melt and a weld.

How Does it Work?
Ultrasonic welding applies small-amplitude high-frequency mechanical vibrations to the parts being joined. The amplitude of these vibrations is from 5 to 400 microns, and the frequency is from 20 to 70 kilohertz, which is above our audible hearing range.Regardless of the application, all ultrasonic welding systems consist of a computer or microprocessor controller; a power supply that is often integrated into the controller; transducer; booster; a sonotrode or horn; and an anvil. The transducer consists of piezoelectric crystals. A DC voltage applied to a piezoeletric crystal causes it to expand or contract, depending on the polarity. In ultrasonic welding, a high-frequency AC voltage-from 20 to 70 kilohertz-from the power supply, causes the crystals to alternately expand and contract, and this is the source of the mechanical vibrations used for ultrasonic welding.
The mechanical vibrations are coupled through the booster to the sonotrode or horn, both of which serve to modify the vibration amplitude to meet specific welding requirements. Parts to be welded are secured between the horn and the anvil by force typically applied by pneumatic or electric servos. The energy of the mechanical vibrations is transmitted to the joint interface from the horn to weld the parts. It is particularly important to note that in welding plastic parts, these vibrations are applied perpendicular to the plane of the joint, while in welding metal parts, the vibrations are applied parallel to the plane of the joint.
The horn supplies all the welding energy to the part and is machined to maximize ultrasonic energy at the joint interface. Ultrasonic plastic welding horns are typically machined from titanium or aluminum alloy. For the most efficient transmission of energy, the end of the horn is designed to fit the geometry of the part it contacts.
Horn design is critical because the mechanical path length must be tuned exactly to the part. Tuning affects weld quality directly and each part design requires a different ultrasonic horn. Correct tuning ensures maximum energy absorption at the joint interface and minimum energy reflection back to the power supply, greatly prolonging equipment life. In welding plastic parts, for example, the length of the horn can be equal to either one-half or one full wavelength of the ultrasonic energy, which is determined by the frequency and the velocity of sound in the horn material.
Three welding modes are typically available, depending on the application. Units can weld by time, energy or distance. For whichever mode is used, an appropriate value of time, distance or energy is programmed into the controller. When the programmed condition is met the ultrasonic energy stops.
Other parameters that affect weld quality and integrity include ultrasonic frequency, force applied to the parts, and amplitude of vibration. Altering any parameter can greatly affect the weld quality. There is no optimal ultrasonic frequency; many units operate near 20 kilohertz.
Ultrasonic welding requires special attention to part dimensions and joint design. In fact, welding parameters are secondary to the part geometry. In ultrasonic plastic welding there are specific joint designs that are dependent on part geometries and material. Ultrasonic metal welding is generally only possible with lap welds and limited thicknesses.
Process physics are complex. Typically large-scale design of experiments or trial and error are used to determine welding parameters. Each material and joint design will behave differently during ultrasonic welding.